Blood Gases (the theory- part 1)
- Christie Roberts
- Jan 29, 2021
- 11 min read
Updated: Jan 22, 2023
This is part one of three (!) posts about blood gases. Right here is a good place to start, and part 2 can be found here with real life practice examples to follow here.

An arterial or venous blood gas (ABG/VBG) is a point of care test that gives rapid information on gaseous exchange, blood pH, haemodynamic stability and electrolytes. In ICU, the majority of patients will have regular ABGs due to the presence of arterial lines for invasive BP monitoring, which give constant easy access to arterial blood. In other settings, such as wards or ED, VBGs will more commonly be used. The main difference is the accuracy of information on gaseous exchange- an ABG will give accurate information on oxygenation of the blood from the partial pressures of Oxygen (PO2). A VBG, by virtue of the fact that venous blood is deoxygenated, won't give an accurate PO2, but other findings are still relevant, and there is a good degree of arteriovenous correlation between ABG and VBG for pH and partial pressure of Carbon Dioxide (PCO2), if the patient is normocapnic, as well as lactate, bicarbonate (HCO3-) and base excess (BE).
If your patient needs arterial stab for a sample (most commonly a DRAP- direct radial artery puncture), please please please use local anaesthetic. An ABG can be incredibly painful, and a little bit of lidocaine goes a long way. If an ABG is needed (for example, in cases of shock, hypotension or respiratory failure, where a VBG may not provide

enough information), the BTS advocates using local unless it is an emergency and use of local would delay getting a result (although use of local can also increase the chance of getting a stab first time, which would surely lead to quicker results...jury's out)- check out the BTS guidance for more info- 1.7.3. Nurses- please advocate for your patients!!!
In this post, I want to give a quick guide to how I read through an ABG. Everybody will have a slightly different process or order that they follow, but having a systematic analysis is a pretty fool-proof way of making sure nothing gets missed. A side note that any values quoted in this post as target ranges or normal values are specific to my Trust and practice, and may vary between Trusts, and equally may vary between individual patients dependent on individual anatomy and physiology.
I loosely follow an A-E approach, covering airway/breathing, circulation, disability and everything else (although there's not really anything under E, it's more of a summary)
Causes are highlighted in pink, treatments in orange.
1. Oxygenation- A/B
First step is always checking if it's venous or arterial. Only an ABG will give you accurate PO2 and SaO2 values. Other than these 2, there is usually decent arterio-venous correlation for values like PCO2, HCO3 and lactate.If PO2 is really low in a patient with O2 sats of 98%, you can assume it's a VBG.
For me, PO2 is usually the first thing I check, especially for a level 3 (intubated and ventilated) patient. PO2 should be above 8kPa, and ideally around 10kPa. If a patient is receiving oxygen and has a PO2 above 10kPa, you can usually wean your O2 down a bit. Goes without saying that if the PO2 is under 8kPa, you'll want to increase your oxygen concentration.
Room air has an FiO2 of 21%- i.e. 21% of the air we breathe in on a regular basis is oxygen, and the other 79% is made up of other gases. In providing oxygen therapy, you can increase the % of oxygen in air delivered. Different flow rates will correspond to different FiO2s, and not all methods are controlled (and therefore have less accurate measures of FiO2). Controlled methods include ventilator oxygen via ETT or trachy, and Venturi masks. When running your gas, you'll be asked to input the FiO2, which is dependent on the oxygen delivery system and flow rate, or in systems such as ventilators or HFNO, the FiO2 will be whatever you've set it at- if not, this table sums up the conversions:

On a ventilator, there are 2 ways of increasing PO2- one is by increasing the FiO2, which can occur in a mandatory or spontaneous ventilation mode (if not ventilated, increase the FiO2 through whichever oxygen delivery method you're using). Alternatively, increasing PEEP High can improve oxygenation by decreasing atelectasis and facilitating alveolar recruitment- but this is only really applicable in mandatory modes such as BiLevel.
The SaO2 on an ABG will also give information about oxygenation. The SaO2 is the oxygen saturation, a measure of how much haemoglobin is combined with oxygen to form oxyhaemoglobin. This will usually correspond with O2 Sats (SpO2) readings from a pulse oximeter, and is generally >94% (in certain patients, 88-92% may be accepted as a target SaO2/SpO2)
2. pH- acidosis or alkalosis? - B/C
The next thing I check is the pH, and the contributing factors- PCO2 and HCO3..
Normal pH is 7.35-7.45. Lower indicates acidosis, higher indicates alkalosis. The nature of the pH shift will be respiratory or metabolic, and this is where CO2 and bicarb come into it.
Carbon dioxide- normal range 4.5-6kPa/ 35-45mmHg- forms carbonic acid in the blood, and is therefore associated with acidosis if it is high. It is removed by breathing- therefore in patient with hypoventilation or low tidal volumes, CO2 can rise. In patients hyperventilating, CO2 will drop, which makes the blood more basic (/alkalotic)
Bicarbonate- normal range 22-26 mEq/L- is a basic molecule which pushes the blood towards alkalosis. It is produced and released by the kidneys, and excreted in urine if not needed. Bodily shifts in bicarbonate are usually slower reactions, so changes to pH will take longer compared to CO2.
Usually, there is an equilibrium that keeps the body's pH in range. The acronym 'ROME' is used to identify the cause of acidosis or alkalosis:

Respiratory Opposite Metabolic Equal.
In respiratory disturbances, the pH will go the opposite way to the PCO2. If in respiratory acidosis (so low pH), PCO2 is high (because there's more carbonic acid in the blood). If PCO2 is low, pH will be high.
Low CO2 leads to RESPIRATORY ALKALOSIS (high pH) and is generally due to hyperventilation (high tidal volumes, and/or a high respiratory rate) Hyperventilation can occur as a consequence of head injury, anxiety/fear/pain, hypoxia (in an attempt to correct low O2, at the expense of losing CO2), COPD exacerbations, acute asthma, and iatrogenic harm in ventilated patients. Patients may present with tachypnoea, tachycardia, light-headedness, decreased serum calcium (so tetany, muscle cramps) and decreased serum potassium (so more tetany, and ECG changes).
The main way to reverse hypocapnia is to decrease respiratory rate or tidal volume (rebreathing CO2 by breathing into a paper bag will work, but isn't usually recommended) and this generally focuses around treating the underlying cause of hyperventilation- this may include anxiolytics, analgesia, supplementary oxygen or ventilatory support for T1RF. Agents that suppress the respiratory drive may be used- including sedatives and opioids. To compensate for respiratory alkalosis, the kidneys will produce less/excrete more bicarb, but levels will usually never drop below 12 mEq/L..
High CO2 leads to RESPIRIATORY ACIDOSIS (low pH) and is conversely caused by hypoventilation leading to a build up of CO2 in the body. Hypoventilation may be due to CNS depression (in head injury, intoxication, anaesthesia), respiratory muscle weakness (in Guillain Barre, ALS, myasthenia gravis), chest wall deformities, morbid obesity, ARDS and unmanaged pain. Patients may present with hypoxia, tachypnoea, dysrhythmias (caused by hyperkalaemia) and mental status changes.

An impaired conscious level can lead to an impaired respiratory drive. This leads to worsening hypoventilation, and subsequent worsening hypercapnia. Severe hypercapnia has an anaesthetic effect and further lowers conscious level and respiratory drive, and a vicious cycle begins.
Opposite to hypocapnia, hypercapnia is addressed by increasing the respiratory rate or tidal volume to blow off more CO2. In many cases of poor ventilation, it may be necessary to take over breathing with intubation and ventilation. Hypercapnia is seen in T2RF, which can be acute, or chronic in CO2 retainers (e.g. COPD). In chronic hypercapnia, care must be taken to not drop CO2 levels and over-oxygenate as this runs the risk of suppressing the hypercapnic respiratory drive- hence target sats of 88-92% in known COPD. Also consider permissive hypercapnia in ARDS presentations, which goes hand in hand with a respiratory acidosis. In the case of pain (where pain on deep inspiration is inhibiting ability to achieve an adequate minute volume), further pain relief will be needed to facilitate deep breathing and higher tidal volumes.
As a compensatory mechanism, the kidneys will produce and retain more bicarb.
Essentially- particularly in a ventilated patient, changing CO2 (and therefore pH) relies on altering the minute volume- the amount of air breathed in 1 minute. Minute volume is calculated by f (respiratory rate) x tidal volume- therefore changing either of these will alter the amount of CO2 expired. RR can be changed in mandatory ventilation modes (e.g. BiLevel) but cannot be controlled in spontaneous modes. Tidal volume is changed by increasing or decreasing pressure support, and this can be done for spontaneous modes (e.g. CPAP/PS). Changes to oxygenation and PCO2 will usually be reflected fairly quickly in the pH.
In metabolic disturbances, the pH will go the same way as the HCO3-. If in metabolic acidosis (so low pH), HCO3- is low (because there's fewer basic/alkaline bicarbonate molecules in the blood). If HCO3- is high, pH will be high, in metabolic alkalosis. Bicarbonate is produced and excreted by the kidneys, so metabolic disorders are often, but not always, related to the renal system.
Low HCO3 leads to METABOLIC ACIDOSIS (low pH), along with conditions causing higher levels of acid products (such as lactate and ketones) in the blood.
All kinds of metabolic acidosis can present with similar symptoms- hyperkalaemia and associated ECG changes, altered respiratory patterns as a form of compensatory hyperventilation (to reduce PCO2), altered mental status and vasodilation with flushed skin.
Low HCO3 can be caused by gastrointestinal HCO3 loss in diarrhoea, renal tubular acidosis (in which either not enough H+ (acid) is excreted in urine, or not enough HCO3 (base) is reabsorbed, leading to excess losses in urine) or renal failure causing decreased production of HCO3. Administration of sodium bicarbonate (NaHCO3) to address acidosis is contentious, and does not have a huge body of evidence demonstrating benefits for morbidity or mortality- in certain situations, NaHCO3 (generally either 1.26% or 8,4%) can be given IV. For info about lactic and ketotic metabolic acidosis, see below...
Lactic acidosis- does what it says on the tin. There's an excess of lactic acid in the blood which drives the pH down. Causes include sepsis and other states of shock or regional tissue hypoperfusion (eg limb ischaemia, post cardiac arrest), or other severe acute illness. An elevated lactate is usually defined as 2.0-2.5mmol/L, while a high lactate is >4mmol/L.. The UK Sepsis Trust counts a lactate of 2 or more as a RED FLAG for sepsis.

In the case of sepsis ,tissues have insufficient oxygen for aerobic respiration, anaerobic respiration is used and lactic acid is produced as a by-product- we all know and love the Sepsis 6, which tells us that a lactate level should be taken within an hour of suspected sepsis .
Lactate clearance can be impacted by severe liver dysfunction- so accompanying hepatic injury in shocked states can further contribute to elevated lactate. Treatment for a high lactate generally centres around reversing hypoperfusion and hypoxia- so fluid volume replacement, inotropes or vasopressors, and supplementary oxygen to maintain O2 sats >94% (remember the sepsis 6!!!)- plus treatment of any underlying conditions/infections.
Ketoacidosis- Acidic ketone bodies (usually acetone, which causes the pear drops breath) are produced in response to low insulin and high glycogen levels. The most common form is Diabetic Ketoacidosis (DKA) which is usually seen in T1D, but occasionally in T2D (10-30% of cases), but alcoholic ketoacidosis (AKA) and starvation ketoacidosis also exist. DKA is generally diagnosed with a pH <7.35, blood glucose >11 and ketones >1.5 , and can present with Kussmaul respirations, signs of severe hyperglycaemia (polydipsia, polyuria, weight loss, vomiting), potassium derangement and mental status/conscious level changes. Treatment involves fluid replacement and insulin therapy, but specific treatment for acidosis is not generally indicated - plus treating any underlying causes, like infection (Abx), poor insulin compliance (psychotherapy/education) or severe illness- MI, pancreatitis, trauma- causing physiologic stress. Also recommended to get your local diabetes team involved early, and monitor K+ as correction of acidosis will shift K+ intravascularly, dropping serum potassium.
High HCO3 leads to METABOLIC ALKALOSIS (high pH).. Symptoms may include N+V and diarrhoea, compensatory hypoventilation to retain CO2, arrhythmias, tachycardia and electrolyte shifts (hypocalcaemia and hypokalaemia).
Causes of high HCO3 can include excessive administration of sodium bicarbonate (iatrogenic harm), or more commonly volume depletion through severe vomiting and polyuria secondary to diuretic use. Low fluid states act as a stimulus for HCO3 reabsorption in the kidneys.
Vomiting will also lead to loss of gastric acid, which further contributes to a shift towards alkalosis.
Metabolic alkalosis can be treated by addressing fluid depletion (IV fluids, at a rate that overcomes hourly sensible and insensible losses), and preventing further losses (e.g. with antiemetics). If it's been caused by giving too much NaHCO3 or diuretics, then stop giving NaHCO3 and diuretics.
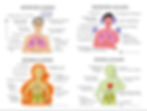
Not my image, but I don't have a reference available for this, sorry!
A note on electrolyte shifts in pH disturbance-
Alkalosis promotes binding of calcium to albumin, which reduces calcium in the blood. Hypocalcaemic symptoms are more commonly associated with respiratory alkalosis compared to metabolic alkalosis.
Increasing pH (alkalosis) leads to a reduction of H+ ions in intracellular fluid. This causes extracellular potassium to move into cells- causing a drop in serum potassium and symptoms of hypokalaemia. The opposite happens in acidosis, leading to hyperkalaemia. Every 10mmHg increase/decrease in PCO2 causes an increase/decrease of 0.5mmol/L K+.
Compensation by the opposing system can sometimes be seen. In these cases, both the PCO2 and HCO3 will be off. First establish the cause of the pH shift (respiratory or metabolic) by seeing which factor matches. Then, establish if the opposing system is attempting to correct the issue.
For example-

this is a RESPIRATORY ACIDOSIS (the pH and PCO2 match). Because the HCO3 is normal but the pH is still out of range, this is UNCOMPENSATED.

this is the same respiratory acidosis but the HCO3 is also out of range. Because the HCO3 is high (attempting to correct the problem by introducing more alkaline molecules) but the pH is still out of range, this is PARTIALLY COMPENSATED.
There's a lot of good resources, particularly on YouTube, that can probably explain pH analysis in much more detail than this, and particularly going into compensation and mixed disorders.
The TL;DR for this very long section is 1) is the pH in range? 2) if not, is it respiratory or metabolic? 3) is the other factor compensating? 4. what can you do to fix the problem?
3. Other pH things- B/C
Base excess (Base (Ecf)) is an accessory to pH analysis, and is a reflector of metabolic pH disturbance. It's officially defined as 'the amount of acid needed to bring 1L of blood to pH 7.4 at a PCO2 of 5.3kPa/40mmHg at 37deg'. The normal range is -2 - +2, where a more negative number indicates the amount of acid that needs to be removed to restore pH (so how much acid is causing a metabolic acidosis, and how much more HCO3- is needed) and a more positive number indicates the amount of acid that needs to be added to restore pH (so how much is needed to correct a metabolic alkalosis, comprised of excess HCO3-).
That concludes part 1 of this series on blood gases, so thank you for reading. My recommendation would be carrying onto part 2, and then having a stab at some examples in part 3. Or you can go straight to part 3, I can't really tell you what to do.
Here's the reference list for this part, helpfully split up into each section so you can find whichever resource is most helpful. As always, fave sources are bolded.
ABG-
Geeky Medics (2020) 'How to take an arterial blood gas (ABG)- OSCE guide' https://geekymedics.com/arterial-blood-gas-sampling/
LITFL (2020) 'VBG versus ABG' https://litfl.com/vbg-versus-abg/
British Thoracic Society (2017' 'BTS guideline for oxygen use in adults in healthcare and emergency settings' in Thorax. 72(51). https://www.brit-thoracic.org.uk/document-library/guidelines/emergency-oxygen/bts-guideline-for-oxygen-use-in-healthcare-and-emergency-settings/
Hudson, T., Dukes, S., Reilly, K. (2006) 'Use of local anaesthesia for arterial punctures' in American Journal of Critical Care. 15(6), pp.595-599. https://www.researchgate.net/publication/6743085_Use_of_Local_Anesthesia_for_Arterial_Punctures
Oxygenation-
British Thoracic Society (2017' 'BTS guideline for oxygen use in adults in healthcare and emergency settings' in Thorax. 72(51). https://www.brit-thoracic.org.uk/document-library/guidelines/emergency-oxygen/bts-guideline-for-oxygen-use-in-healthcare-and-emergency-settings/
pH-
Moon, H., Lee, S., Chung, J., Bum, C. (2011) 'Hypocalcemia and hypokalemia due to hyperventilation syndrome in spinal anesthesia- a case report' in Korean Journal of Anesthesiology. 61(6), pp. 519-523. https://www.ncbi.nlm.nih.gov/pmc/articles/PMC3249577/#B8
Wiseman, A., Linas, S. (2005) 'Disorders of potassium and acid-base balance' in American Journal of Kidney Diseases. 45(5), pp. 941-949. https://www.ajkd.org/article/S0272-6386(05)00284-2/pdf
Sharma, S., Hashmi, M. (2020) 'Hypocarbia'. https://www.ncbi.nlm.nih.gov/books/NBK493167/
Farkas, J. (2017) 'PulmCrit – Optimizing the respiratory drive to avoid failure' https://emcrit.org/pulmcrit/respiratory-drive/
EmCrit is a lovely resource which frankly, I don't use enough. This PulmCrit page has plenty of pictures (love love love)
Andersen, L., Mackenhauer, J., Roberts, J., Berg, K., Cocchi, M., Donnino, M. (2013) 'Etiology and therapeutic approach to elevated lactate' in Mayo Clinical Proceedings. 88(10), pp. 1127-1140. https://www.ncbi.nlm.nih.gov/pmc/articles/PMC3975915/
Hechanova, L. (2020) 'Renal Tubular Acidosis (RTA)' in MSD Manual. https://www.msdmanuals.com/home/kidney-and-urinary-tract-disorders/disorders-of-kidney-tubules/renal-tubular-acidosis-rta
The UK Sepsis Trust (2018) 'Sepsis Six Pathway' https://sepsistrust.org/wp-content/uploads/2018/06/ED-adult-NICE-Final-1107.pdf
Burger, M., Schaller, D. (2020) 'Metabolic acidosis' https://www.ncbi.nlm.nih.gov/books/NBK482146/
NHS (2020) 'Diabetic Ketoacidosis' https://www.nhs.uk/conditions/diabetic-ketoacidosis/
Ghimire, P., Dhamoon, A. (2020) 'Ketoacidosis' https://www.ncbi.nlm.nih.gov/books/NBK534848/
BNF (2021) 'Sodium bicarbonate' https://bnf.nice.org.uk/drug/sodium-bicarbonate.html
Ghauri, S., Javaeed, A., Mustafa, K., Podlasek, A., Khan, A. (2019) 'Bicarbonate therapy for critically ill patients with metabolic acidosis: a systematic review' in Cureus. 11(3), p4297. https://www.ncbi.nlm.nih.gov/pmc/articles/PMC6538112/
Lewis, J. (2020) 'Metabolic acidosis' in MSD Manual. https://www.msdmanuals.com/en-gb/professional/endocrine-and-metabolic-disorders/acid-base-regulation-and-disorders/metabolic-alkalosis
GeekyMedics (2021) 'ABG interpretation' https://geekymedics.com/abg-interpretation/
Baid, H., Creed, F., Hargreaves, J. (2016) Oxford handbook of critical care nursing. 2nd Edn. Oxford University Press, Oxford